Methane Satellite Missions Capability Comparison
Marketing
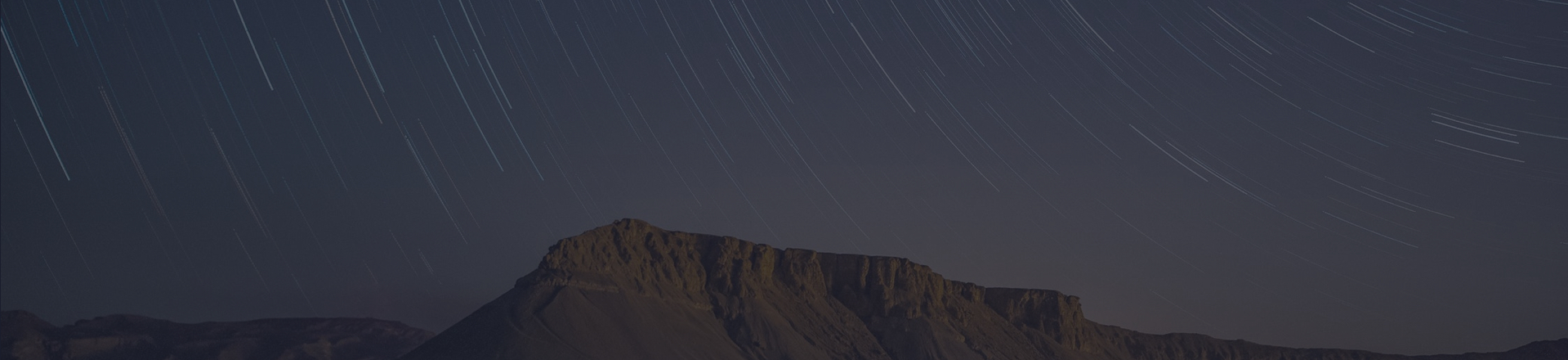
Introduction
MethaneSat, the newest satellite with methane monitoring capabilities was launched into space in March 2024. The launch has generated a new wave of interest in methane monitoring via satellite. The US non-profit Environmental Defence Fund (EDF) developed the satellite with the New Zealand Space Agency. The data will be publicly available for free and is designed to call out super-emitters of methane gas.
Several different types of satellites exist for monitoring different types of methane emissions. MethaneSat won’t be the newest satellite in space for long, with several more planned for launch in the next few years. This increasing data availability makes for an exciting time to investigate the opportunities these types of satellites bring for monitoring and regulating methane emissions.
This article provides a brief overview of the capabilities of some current satellites with methane monitoring capabilities, as well as those planned for launch, and how they compare.
Observing Methane from Space
The shortwave infrared (SWIR) part of the electromagnetic spectrum is important for the detection of methane. Some satellites are designed specifically to detect methane e.g. GHGSat WAF-P and MethaneSat, with very specific measurements in the SWIR, these are the focus of this article. Multispectral sensors with multiple bands across wavelengths including the capture of information in the SWIR e.g. Worldview-3 and Sentinel-2 can also be used to detect methane.
Methane-detecting satellite sensors can be broadly separated into two categories:
These are two broad categories, with a range of capabilities within each. The different types can be used in combination, using the global mappers to identify hot spots, with the facility scale monitors then able to identify emitters. A demonstrated example is at a landfill site in Madrid, investigated by SRON Netherlands Institute for Space Research and GHGSat, using a range of satellite sensors to analyse emissions.
More satellites in orbit improve the capability to image a site at a higher frequency with multiple sensors which is important for large-scale high pollution events. Though for measurements to be directly comparable more work is needed on interoperability and standardisation. The Committee for Earth Observation Satellites (CEOS) is key to this, coordinating the different operators together from across the World.
Current and Future Satellite Missions
The below graphic shows the current and upcoming methane-specific satellite sensors and the category into which they fit according to CEOS.
Methane Observing Satellites accessed via CEOS Greenhouse Gas Satellite Missions Portal [Source: acp.copernicus.org, Credit: GeoSapient]
The below table provides information on a selection of the sensors currently available and planned for launch, to allow a comparison of capability, ordered by spatial resolution. The information has been taken from a combination of sources, the CEOS Greenhouse Gas Satellite Mission Portal, Jacob et al. (2002), Global Methane Tracker 2024 and the EO Portal.
This table displays some of the core information about some of the methane-specific missions’, in descending order by spatial resolution. The information is sourced from multiple locations including CEOS (1), Jacob et al. (2022) (2), Global Methane Tracker 2024(3) and EO Portal(4). Conflicting and outdated information exists between sources and this table is a best effort to provide consolidated and updated information as of April 2024. The source referenced for each number is shown by the superscript reference. Many of these sensors are yet to be launched and so exact capabilities are yet to be tested.
The choice of the most appropriate sensor is dependent on the asset or environment to be measured and how the information is to be used. Aspects to consider and how they relate to the table include:
There are further characteristics available on each of these sensors which should be taken into account when choosing a sensor such as precision and error rates.
Data Access
Some of the sensors listed are yet to be launched, others have data ready to use with data available on various portals.
Methane Monitoring Data Supply for UK Programme
This article has been written as part of the methane monitoring data supply for the UK Programme.
UK Organisations can request access to the methane dataset for R&D purposes and explore how the methane dataset could support their GHG reduction targets.
Visit the webpage to find out more: sa.catapult.org.uk/projects/methane-monitoring/
Email: CH4.data@sa.catapult.org.uk
References
Jacob, D.J., Varon, D.J., Cusworth, D.H., Dennison, P.E., Frankenberg, C., Gautam, R., Guanter, L., Kelley, J., McKeever, J., Ott, L.E. and Poulter, B., 2022. Quantifying methane emissions from the global scale down to point sources using satellite observations of atmospheric methane. Atmospheric Chemistry and Physics, 22(14), pp.9617-9646.
This blog was written in April 2024