Monitoring Methane Emissions from Space
Marketing
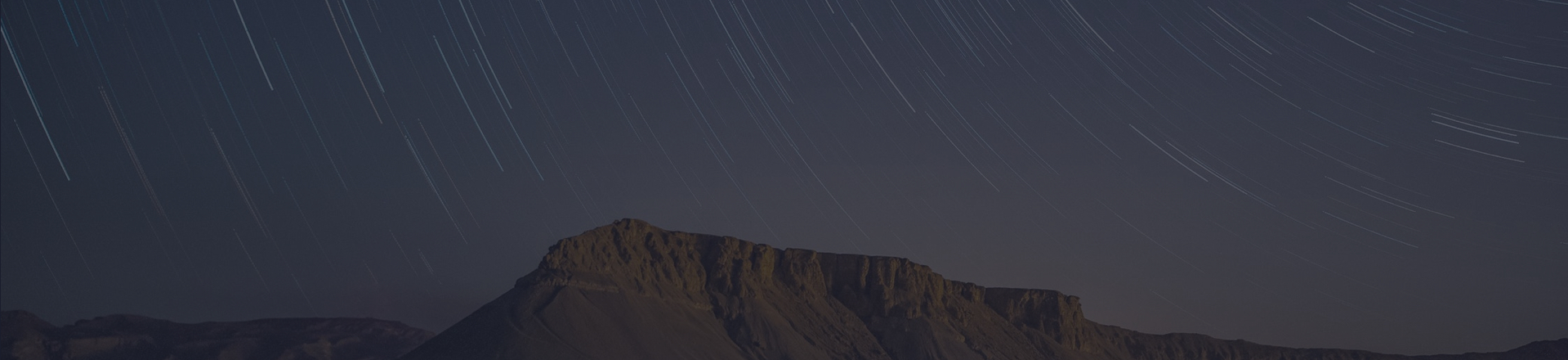
The Satellite Applications Catapult, in collaboration with the Ordinance Survey (OS) and the Department for Environment, Food, and Rural Affairs (DEFRA) have curated a new methane monitoring dataset, using satellite observation data from GHGSat funded by the UK Space Agency (UKSA). This initiative offers a unique opportunity for organizations in the UK, spanning commercial, governmental, and research sectors, to explore innovative applications of space-based methane observations at the asset level. This blog post highlights the significance of methane and discusses GHGSat’s methane monitoring capabilities, as well as how you can access a subset of this valuable data through our exciting program.
Methane is a potent greenhouse gas whose global mean concentration reached 1.92 ppm in 2023, an increase of 16% over the past 4 decades (NOAA). While CH4 concentrations are significantly smaller than CO2 (423.68 ppm in 2023 – NOAA), its molecular structure makes it far more effective at trapping reflected thermal radiation, with a greenhouse potential ~85 times higher than CO2 over a 20 year cycle (IPCC 6th Assessment Report 2022). Accordingly, CH4 constitutes 19% of the combined effective radiative forcing (Myhre et al., 2013), with well mixed methane (chemical alterations to atmospheric ozone and halocarbons) providing a ERF of 1.19 [0.81 to 1.58] W m-2 (relative to 1750), second only to CO2 (3.9 ± 0.5 Wm–2) (Fig.1).
Figure. 1: Climate change through effective radiative forcing (ERF) described by a) emitted contributions to ERF, estimated by the Coupled Model Intercomparison Project Phase 6 (CMIP6) models( 5–95% error bars showing uncertainties); and b) estimates of the net cumulative energy change (ZJ = 1021Joules) for the period 1971–2018 by all components, net forcing described by dashed black line. Source IPCC Report 2022
The global climate crisis requires states and industries to take rapid action to alter the trajectory of anthropogenic induced climate change. Reducing methane emissions is key to that target, as with a relatively short atmospheric lifetime of ~10 years (CO2 =300-1000 years), changes in the volume and frequency of methane emissions will have a significant effect on the near-term warming rate (Johannsson et al., 2008).
Methane emissions occur globally, with many anthropogenic point sources originating from several key industries, including
Studies have shown, that between 60-90% of methane leaked from anthropogenic sources originate from a relatively small fraction of events (~5%), each producing flux (𝑄) in excess of 100 kg hr-1 (Duren et al., 2019).
The unique monitoring capabilities of earth observation (EO) satellites provide the only means to regularly monitor methane emissions at a global scale. Global missions, such as the TROPOspheric Monitoring Instrument (TROPOMI), aboard Copernicus’ Sentinel-5p satellite, and the Greenhouse Gases Observing SATellite (GOSAT) (Fig. 2) have provided regional maps of methane concentrations since 2017. Through these medium resolution (> 7km) daily maps, we have begun to understand where large concentrations of methane regularly occur. Yet, to better understand the causes of these emissions, finer resolution, targeted observations are required.
Figure 2: Example methane emission measuring mission across north America using Greenhouse Gases Observing SATellite (GOSAT), observing methane emissions with a spatial resolution of 10 km. These types of data have improved our understanding large-scale methane emissions; however, the resolution is too coarse to resolve facility scale sources of emissions. Source: NASA EarthData
GHGSat’s constellation of nine (eleven by the end of 2023) methane monitoring satellites operate in a 500 km polar orbit, with the potential for daily monitoring of tasked target locations. The wide-angled Fabry Perot (WAF-P) imaging spectrometer has a spatial resolution of 25m and an image footprint of 12 km2, providing both the resolution detail to define facility-scale features and the spatial scale to observe the full extent of the plume. This method has a detection threshold of CH4 >100 kg/hr above background emissions, within optimal wind conditions (<3 m/s).
By describing the total downwind emission plume, these data compliment stationary ground-based systems, providing industry stakeholders and policy makers the ability to accurately monitor total methane emissions from point (chimney or vent) and diffuse (landfill or open-pit mine) sources.
The satellite imaging spectrometer operates in the shortwave infrared (SWIR) between 1630 – 1675 nm where methane, CO2, and water vapour gases are known to absorb reflected solar radiation. The atmospheric spectral absorption characteristic of a given location are constructed by multiple (~200) overlapping images, taken as the satellite passes over the target.
To translate these absorption profiles to methane concentrations, these data are first compared to background atmospheric gases, including methane (CH4), carbon dioxide (CO2), and water vapour, with the residual difference converted into methane concentrations per pixel. Here, the measured absorption profile of the atmosphere can be quantified as number of methane molecules observed (mol m-2)) (Fig. 3), converted to parts per billion volume (ppbv) above background emission (Fig. 3).
Figure 3: Example of GHG methane products over Dawson Mine, Australia, August 2022, including a) the observation field of view footprint, b) the full abundance product, and c) the extracted emission plume data. Emission values present a parts per volume against background methane concentrations. Top) image of the Dawson Mine. Digital Image. Heather Jenkins https://www.google.com/maps/place/Dawson+Mine
A full 12 km scene is compiled of around 200,000 ground cell (pixel) profiles and is presented as an abundance image (Fig. 3.b). These are assessed using AI and validated by trained operators to identify emission plumes. Finally, the emission plume shape and local wind conditions are analysed, deriving an emission source rate and an estimate origin of the emission.
Through the methane monitoring project, eligible users will have access to a large subset of GHGSat data between January 2021 and August 2023. This dataset covers a range of sites selected across multiple industries around the globe and includes all GHGSat archive observations for the UK (Fig. 4).
In total, 1,674 satellite observations across 424 specific sites were selected, including 2,057 individually identified emission plumes (e.g., Fig. 3.c) and 512 full scene (12 km2) background methane abundance data (e.g., Fig. 3.b) – 60 of these include emission products.
Figure 4: UKRI methane emission monitoring sites of interest, showing a) distribution of selected GHGSat methane emission and abundance concentration data, and b) site locations by industry sector.